NGS, qPCR, or Sanger Sequencing: An Assay Selection Guide
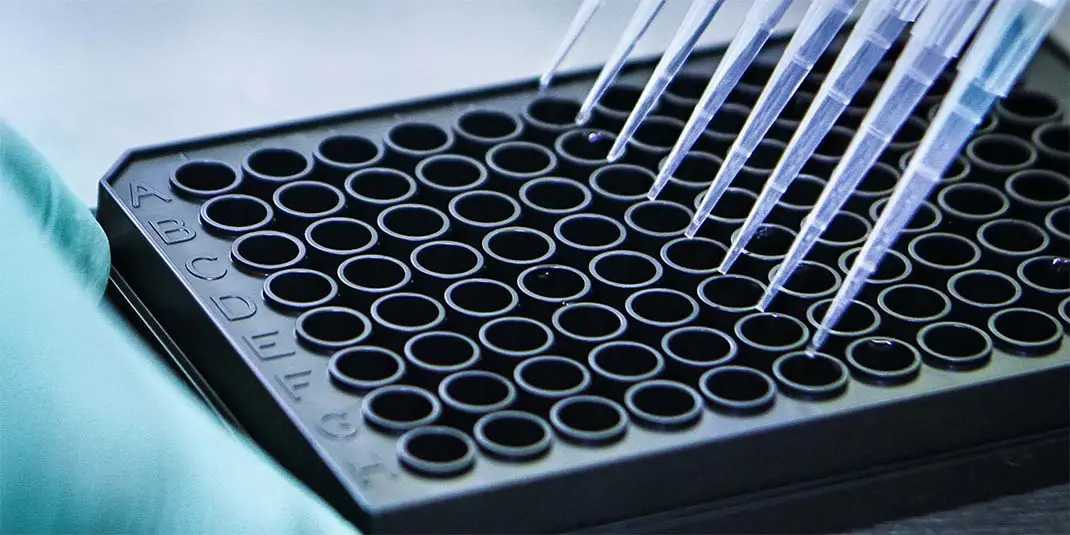
Interactive Assay Selection Tool
Today’s molecular biologists have many options to interrogate their genome or transcriptome of interest. Next generation sequencing (NGS), quantitative PCR (qPCR), digital PCR (dPCR), and Sanger sequencing are used widely in genomics; however, choosing the best tool for your project isn’t always intuitive. For example, these four technologies provide valuable insights into specific transcripts: qPCR evaluates expression levels, PCR + Sanger identifies sequences, dPCR quantifies or distinguishes low-abundance targets for absolute quantification, and NGS accomplishes a range of these tasks. There are several factors to consider, but most important are the objectives of your experiment. It is imperative that the assay type is well-suited to answer your biological question. To learn more about the method best suited for your project goals, use our interactive selection guide as well as the practical information about PCR + Sanger, qPCR, dPCR, and NGS approaches below.
PCR and Sanger Sequencing
Sanger sequencing is the gold standard for DNA sequencing. This method relies on in vitro polymerization using fluorescent dye terminators and capillary electrophoresis to separate and detect the sequencing products. It examines the sequence of the resulting DNA fragments, integrating the sequencing data from all products to reconstruct the complete DNA sequence. It can be paired with PCR to interrogate regions of the genome or transcriptome in a relatively fast two-step process; PCR bulks up the target DNA in sufficient quantity to be analyzed by Sanger sequencing. Genomic DNA cannot be analyzed directly by Sanger sequencing, as the technology requires a high number of copies of the target sequence.
Input sample: Purified genomic DNA or cDNA. A region of interest, usually less than 1 kb, will be amplified via PCR, purified, and then sequenced. Sanger sequencing requires a homogeneous template for best results, so the PCR assay should be optimized to eliminate off-target amplification.
What can be targeted: A region of the genome or a transcript. Each Sanger sequencing reaction usually provides at least 500 bp of high-quality data. Amplicons larger than 500 bp can be fully analyzed using multiple sequencing reactions. As both PCR and sequencing rely on primers, the locus of interest (or more precisely, the primer binding sites) must be defined beforehand. However, the sequence between the primers can be unknown, enabling sequence discovery.
Number of targets: 1 per assay. No multiplexing is possible with the PCR + Sanger method. Only one target is amplified per PCR reaction per sample, and the resulting product is used for one or more sequencing reactions.
Output data: Chromatograms (also known as trace or AB1 files) are used to determine the sequence of the PCR product (in a SEQ or FASTA file). If more than one sequencing reaction is performed per amplicon, the data can be assembled into a contiguous sequence. Sanger sequencing can detect a certain degree of heterogeneity, but it doesn’t provide quantitative information. For example, a single nucleotide polymorphism (SNP) from a heterozygous individual would appear as a mixed base in the chromatogram—that is, two overlapping peaks at the same position. The data quickly becomes less interpretable with greater diversity in the amplicon population. Amplification and Sanger sequencing of the 16S rRNA gene from a complex microbial community would produce an indecipherable chromatogram of overlapping traces.
Advantages:
- Rapid sample to answer
- Sequence confirmation and discovery
- Low per-sample cost
Disadvantages:
- Not quantitative
- Low scalability
Quantitative PCR (qPCR)
Polymerase chain reaction (PCR) amplifies a target region in an exponential manner. By measuring the amount of newly synthesized DNA after each cycle, the starting amount of target DNA/RNA can be calculated. Quantitative PCR (qPCR), also known as real-time PCR, uses fluorescence-based imaging to measure the amount of amplified DNA in real time during the reaction. The fluorescent label can be an intercalating dye, such as SYBR™, that preferentially binds to double-stranded DNA or a probe—that is, a modified oligo with an attached fluorophore and quencher.
Input sample: Purified genomic DNA or complementary DNA (cDNA). For gene expression analysis, total RNA is isolated and converted into cDNA via reverse transcription (RT). It’s possible to perform cDNA synthesis and qPCR amplification in the same tube, a method known as one-step RT-qPCR, which reduces sample handling.
What can be targeted: A region of the genome or a transcript. Typically, a region of 70-200 bp will be amplified, although longer amplicons are possible. The PCR primers and probe, if applicable, provide target specificity. As these oligos must be designed and synthesized prior to performing the assay, qPCR is limited to targeting known sequences.
Number of targets: 1 to 5 per reaction. Multiplexing is possible with multiple probes, each targeted to a unique sequence and conjugated to a different reporter dye.
Output data: The quantification cycle (Cq), also known as the threshold cycle (Ct), is the key result, calculated from the raw data – the plot of fluorescence over time. It represents the cycle number where the signal from amplification exceeds background fluorescence. A lower Cq value correlates with a higher amount of the input target sequence, such as higher gene expression for RNA samples. Quantitative PCR can also be used as a highly sensitive and reproducible detection assay with a well-defined limit of detection.
Advantages:
- Quantitative
- Rapid sample to answer
- Multiplexing possible
- Low per-sample cost
Disadvantages:
- No sequence discovery
- Low scalability
Digital PCR (dPCR)
Digital PCR (dPCR), or digital polymerase chain reaction, is a highly precise molecular biology technique used to quantitatively analyze and amplify DNA or RNA samples. Unlike traditional qPCR, which measures DNA amplification in a continuous manner, dPCR divides the sample into thousands of individual partitions, each containing either zero or one target molecule. By isolating these discrete units, dPCR provides an absolute quantification of the target molecules, offering exceptional sensitivity, accuracy, and resistance to PCR inhibitors. This makes dPCR especially valuable in applications where precise quantification of rare genetic material, copy number variation, or absolute quantification is required, such as in cancer research, viral load determination, and environmental DNA analysis.
Input sample: Purified genomic DNA or complementary DNA (cDNA).
What can be targeted: A region of the genome or a transcript. Typically, a region of 70-200 bp will be amplified, although longer amplicons are possible. The PCR primers and probe, if applicable, provide target specificity. As these oligos must be designed and synthesized prior to performing the assay, dPCR is limited to targeting known sequences. It is particularly useful for applications that require accurate measurement of nucleic acid concentrations, detection of rare mutations, and absolute quantification of targets.
Number of targets: 1 to 5 per reaction. Multiplexing is possible with multiple probes, each targeted to a unique sequence and conjugated to a different reporter dye.
Output data: The output data of dPCR consists of quantitative information about the target nucleic acid molecules present in the analyzed sample. Unlike traditional qPCR, where the output is often presented as cycle threshold (Ct) values, dPCR provides absolute quantification of the target molecules.
Expected dPCR experiment outputs are:
- Positive, negative, and total partitions
- The number of target molecules per unit volume
- Absolute copy number of the target based on the partition data
Advantages:
- Absolute quantification
- High sensitivity
- Rare mutation detection
- Robust to PCR inhibitors
- No need for a standard curve
- Accurate detection of small fold changes or low abundance targets
Disadvantages:
- Fewer established assays compared to qPCR
- High sensitivity assays may require optimization
- Expertise in assay design is essential for producing meaningful data
Next Generation Sequencing (NGS)
Next generation sequencing (NGS) encompasses several technologies and techniques that enable high-throughput sequence analysis. It provides both qualitative and quantitative data, combining the advantages of qPCR and Sanger sequencing. It can be used to comprehensively examine the entire genome/transcriptome or deployed in a targeted manner to analyze one or a few loci. The general strategy involves creating a library of DNA molecules, often large in number and diverse, with common flanking sequences containing binding sites for universal sequencing primers. The molecules are then sequenced in parallel, the details of which depend on the platform used.
Input sample: Genomic DNA or total RNA.
What can be targeted: Some or all the genome/transcriptome. Whole genome sequencing and RNA sequencing (RNA-Seq) are comprehensive and unbiased methods to profile the genome and transcriptome, respectively. Many targeted NGS methods are available and may involve using hybridization baits or PCR for selection of one to thousands of loci prior to sequencing.
Number of targets: 1 to >10,000.
Output data: Raw data as FASTQ files. Bioinformatics is used to assemble sequences, calculate read counts, and perform downstream analysis (e.g., differential gene expression).
Advantages:
- High throughput
- Unbiased sequence discovery
- Flexible assay design
- High multiplexing possible
- Low per-base cost
Disadvantages:
- Slower than qPCR or PCR + Sanger
- Higher per-sample cost (although it depends greatly on the specifics of the NGS assay)
Comparison of Assays
PCR + Sanger | qPCR | dPCR | NGS | |
---|---|---|---|---|
Quantitative | No | Yes | Yes | Yes |
Sequence Discovery | Yes | No | No | Yes |
Number of Targets Per Reaction | 1 | 1 to 5 | 1 to 5 | 1 to >10,000 |
Target Size | ~500 bp per sequencing reaction per PCR product | Typically 70 to 200 bp | Typically 70 to 200 bp | Up to >100 Gb |
Run Time | PCR: 1-3 hours Sanger sequencing: ~8 hours |
1-3 hours | 1-3 hours | Library prep: Hours to days Sequencing: Hours to days |
Relative Cost Per Reaction | $ | $ | $$ | $$ to $$$$ |
Common Applications | • Variant/mutation analysis • Genotyping • CRISPR editing analysis • Bacterial/fungal identification • Confirmatory sequencing |
• Gene expression analysis • Copy Number Variation (CNV) analysis • Pathogen detection and quantification • Confirmatory testing for NGS |
• Absolute copy number variation (CNV) • Rare mutation and allelic discrimination • Low-abundance transcript detection • Viral titer • Circulating tumor DNA (ctDNA) and cell-free DNA (cfDNA) detection in liquid biopsy |
• Gene expression profiling • Whole genome assembly and analysis • Genome annotation • Variant discovery and analysis • CRISPR editing analysis |
Advantages | • Rapid sample to answer • Sequence confirmation and discovery • Low per-sample cost |
• Quantitative • Rapid sample to answer • Multiplexing possible • Low per-sample cost |
• Absolute quantification • High sensitivity • Rare mutation detection • Robust to PCR inhibitors • No need for a standard curve • Accurate detection of small fold changes or low abundance targets |
• High throughput • Unbiased sequence discovery • Flexible assay design • High multiplexing possible • Low per-base cost |
Disadvantages | • Not quantitative • Low scalability |
• No sequence discovery • Low scalability |
• Low scalability • Higher cost compared to qPCR |
• Slower • Higher per-sample cost |
Key Takeaways
- qPCR or dPCR is used to rapidly quantify DNA/RNA:
- PCR + Sanger sequencing is recommended for sequence confirmation/discovery of short regions of the genome or transcriptome
- NGS is ideal for examining larger parts of the genome/transcriptome and analyzing complex samples as it provides high-throughput sequencing and quantification of both known and unknown sequences
Conclusion
With so many genomics tools available today, it can be difficult to choose which assay suits your project. By considering a few factors, you can quickly zero in on the best solution for your application. The guidelines in this article are by no means exhaustive, but they provide a good starting point when designing your genomics project.
Contact one of our experts to discuss which method may be best suited for your research.
Daily Insight
Recent Posts
Recent Post Tags
- next generation sequencing
- gene synthesis
- rna sequencing
- transcriptomics
- sanger sequencing
- aav-itr
- itr sequencing
- proteomics
- single-cell sequencing
- aav plasmid prep
- antibody discovery
- cell and gene therapy
- olink
- quantitative pcr (qPCR)
- rna therapeutics
- PacBio
- circular rna (circRNA)
- digital pcr (dPCR)
- lentivirus
- long-read sequencing
- mRNA
- oxford nanopore sequencing (ont)
- pcr + sanger sequencing
- whole plasmid sequencing